As the conversations around hydrogen as a key to decarbonising our lives accelerate, sometimes here at Ryze we can get geeky.
And when a seriously bright team from the US develop a succesful new method of converting seawater into hydrogen fuel, we think it’s ok to go a little deeper into the science, because it’s both important and fascinating.
SLAC Stanford researchers have developed a new system for turning seawater in hydrogen fuel. The outcomes of their research may aid in the production of low-carbon fuel for electric grids, automobiles, boats, and other types of infrastructure.
Seawater is essential for life on our planet due to its composition of hydrogen, oxygen, sodium, and other elements. However, the intricate chemical makeup of seawater has made it challenging to extract hydrogen gas for use in clean energy applications.
The group of scientists from the SLAC National Accelerator Laboratory and Stanford University, in collaboration with the University of Oregon and Manchester Metropolitan University, have developed a method to extract hydrogen from seawater by chanelling it through a dual-membrane system using electricity.
Their inventive system effectively generated hydrogen without significant production of detrimental by-products. The research findings, featured in Joule, have the potential to promote the development of low-carbon fuels.
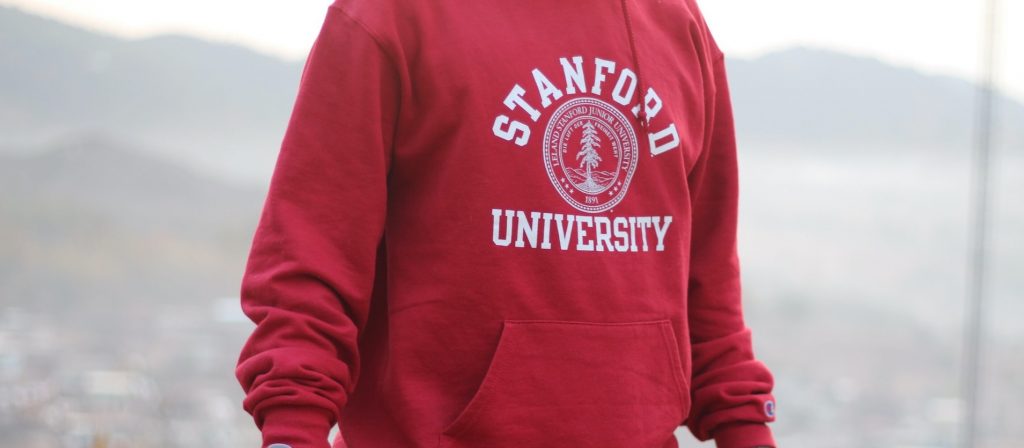
A group of scientists from the SLAC National Accelerator Laboratory and Stanford University, in collaboration with the University of Oregon and Manchester Metropolitan University, have developed a method to extract hydrogen from seawater by chanelling it through a dual-membrane system using electricity.
“Many water-to-hydrogen systems today try to use a monolayer or single-layer membrane. Our study brought two layers together.” Said associate staff scientist with the SUNCAT Center for Interface Science and Catalysis, a SLAC-Stanford joint institute, Adam Nielander.
“These membrane architectures allowed us to control the way ions in seawater moved in our experiment.”
The majority of hydrogen production efforts begin with freshwater or desalinated water, which can be costly and energy-intensive. However, processed water is more straightforward to work with since it contains fewer chemical elements or molecules floating around.
The researchers stated that water purification is costly, energy-consuming, and increases the intricacy of equipment. Additionally, natural freshwater, which is also a limited resource on Earth, contains numerous impurities that are problematic for modern technology.
SLAC and Stanford postdoctoral researcher Joseph Perryman, said: “To work with seawater, the team implemented a bipolar, or two-layer, membrane system and tested it using electrolysis, a method that uses electricity to drive ions, or charged elements, to run a desired reaction.
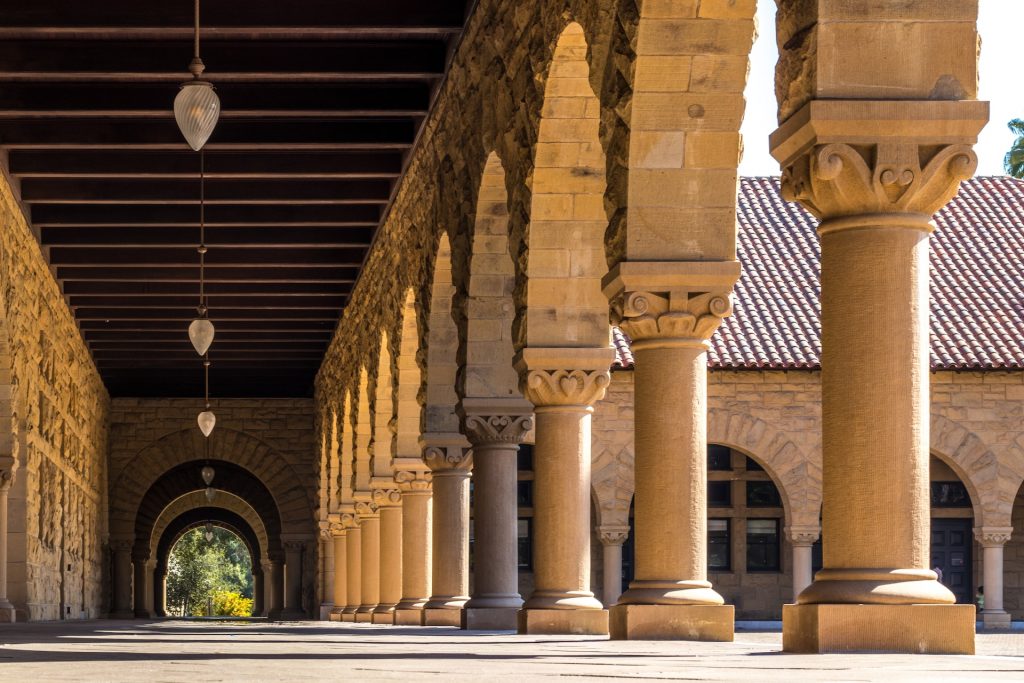
Pictured: Stanford University
They started their design by controlling the most harmful element to the seawater system – chloride.
There are many reactive species in seawater that can interfere with the water-to-hydrogen reaction, and the sodium chloride that makes seawater salty is one of the main culprits,” Perryman said.
In particular, chloride that gets to the anode and oxidizes will reduce the lifetime of an electrolysis system and can actually become unsafe due to the toxic nature of the oxidation products that include molecular chlorine and bleach.”
The bipolar membrane in the experiment allows access to the conditions needed to make hydrogen gas and mitigates chloride from getting to the reaction center.
Perryman continued “We are essentially doubling up on ways to stop this chloride reaction.”
The primary functions of an optimal membrane system include separating hydrogen and oxygen gases from seawater, selectively transporting useful hydrogen and hydroxide ions while inhibiting other seawater ions, and avoiding undesirable reactions. Achieving these three functions simultaneously is a difficult task, and the team’s research aims to investigate systems that can effectively integrate all three requirements.
During their experiment, the team observed that positive hydrogen ions, known as protons, passed through one of the membrane layers to reach a collection point, where they could be converted into hydrogen gas by reacting with a negatively charged electrode or cathode. The second membrane in the system solely permitted negative ions, like chloride, to travel through.
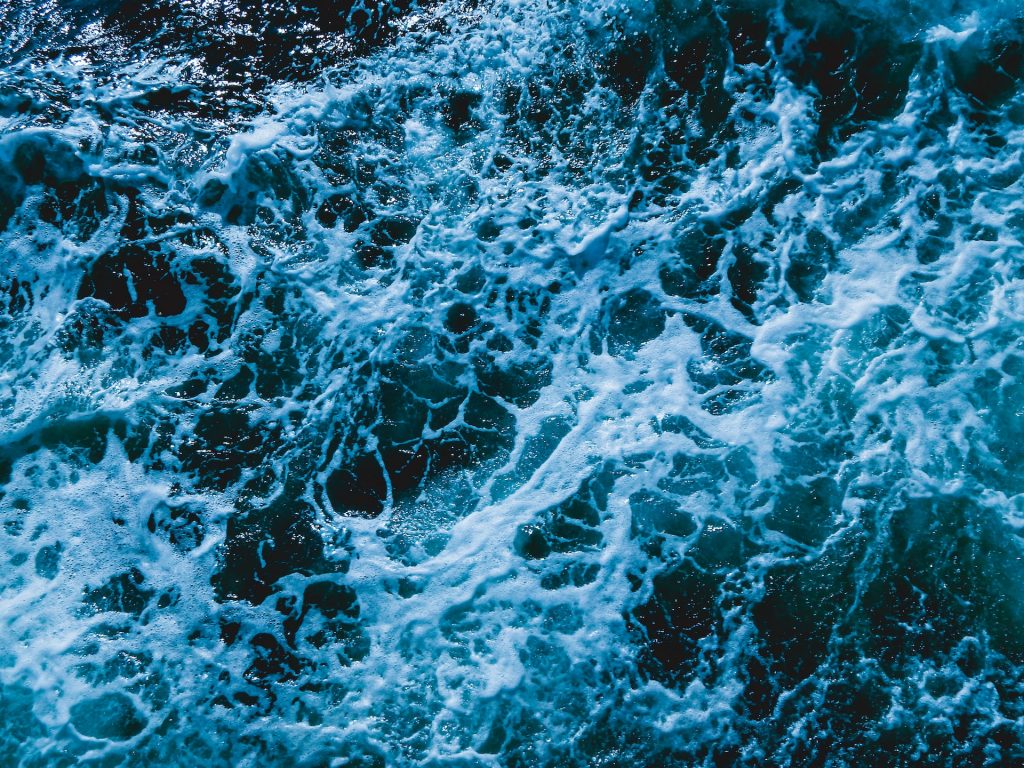
There are many reactive species in seawater that can interfere with the water-to-hydrogen reaction, and the sodium chloride that makes seawater salty is one of the main culprits.
To further prevent negative ions from moving to unwanted areas, a membrane layer with fixed negatively charged groups was also utilised, according to co-author Daniela Marin, who is a graduate student in chemical engineering at Stanford University.
The membrane with a negative charge demonstrated remarkable efficiency in preventing the passage of chloride ions during the team’s experiments, and their system functioned without creating hazardous by-products such as bleach or chlorine. The study not only aimed to develop a membrane system for producing hydrogen from seawater but also contributed to a more comprehensive comprehension of how seawater ions interact with membranes, which could assist researchers in creating sturdier membranes for various applications, including the production of oxygen gas.
The team’s next step is to enhance their electrodes and membranes by employing materials that are more abundant and easier to extract. This design modification could simplify the scaling of the electrolysis system to produce hydrogen on a larger scale, which is crucial for energy-intensive industries such as transportation, according to the team.
“The future is bright for green hydrogen technologies. The fundamental insights we are gaining are key to informing future innovations for improved performance, durability, and scalability of this technology.” said Thomas Jaramillo, professor at SLAC and Stanford and director of SUNCAT
The project is supported by the Stanford Doerr School of Sustainability Accelerator, the U.S. Office of Naval Research, the DOE’s Energy Efficiency and Renewable Energy Fuel Cell Technologies Office, and the DOE’s Office of Basic Energy Sciences, Chemical Sciences, Geosciences, and Biosciences Division via the SUNCAT Center for Interface Science and Catalysis, a joint institute of SLAC and Stanford.
To learn more about Ryze Hydrogen, click here.